Technical Analysis
Introduction
The following section will go into the technical analysis of the implementation of solar energy on Lafayette College’s Campus. The analysis will aim to provide a technical background on the usage of solar energy as a renewable energy source. The section analyzes two different types of solar power, how they function, noticeable differences and then ultimately provides Lafayette with a recommendation for which panel would be most optimal at Lafayette College. Next, the section will look into the returns on energy from solar panel usage. Through calculations, quantifiable metrics on the amount of energy that can be used by facilities on campus will be determinable. Next, six different potential locations will be analyzed for housing solar energy. Several variables must be met in order for a building to be able to hold solar panels. Lastly, the research will focus on three locations on campus and how these locations meet the criteria needed to house solar energy.
Solar Panel Analysis
Every year the Earth receives around 1.5×1018 kWh of solar energy, making it by far the most abundant energy resource available to mankind in our existence (Crawley, 2016). One of the ways the energy of the sun can be harvested and then used is through photovoltaic panels. A French physicist, Alexandre-Edmond Becquerel, discovered photovoltaic panels in 1839 (Crawley, 2016). Since then much advancement has been made in photovoltaic technology and currently it is one of the most widely used methods to harvest energy from the sun. A photovoltaic cell works by directly transforming solar radiation into electrical energy through the PV effect without any thermal cycles, mechanical cycles or chemical reactions (Crawley, 2016). The photovoltaic effect or the PV effect occurs when two dissimilar materials in close contact produce an electrical voltage when under contact of radiant energy (Encyclopedia Britannica, n.d.). A photovoltaic panel is separated into two silicon layers, the top layer with phosphorus to create a negative charge and the bottom layer with boron to decrease the amount of electrons to create a positive charge (Newton, 2015). When a photon hits the panel an electron is knocked out of the silicon junction. This electron is then collected with a metal conductive plate and then can flow like any other energy source (Crawley, 2016). Photovoltaic is known as one of the most effective forms of solar energy but there is another alternative that could be feasible to implement at Lafayette College.
Solar thermal is another form of solar energy that can be used as a renewable energy source. Solar thermal concentrates solar radiation as a source of heat to generate a hot fluid for a downstream energy conversion process (Crawley, 2016). Solar thermal can be used to produce electricity, solar fuels, or directly use processed heat (Crawley, 2016). In solar thermal systems the absorbed radiation will be turned into heat by the excited electrons falling back to their original energy level through energy transfers to the surrounding atoms (Mackay, 2015). In order to fully utilize solar thermal the incorporation of thermal storage is required. Through solar thermal, the suns energy can be leveraged to heat water that can then be used in a building for hot water. Furthermore, solar thermal can be particularly effective for buildings that require large amounts of hot water. Solar heaters for swimming pools are the most practical application (Lunde, 1980).
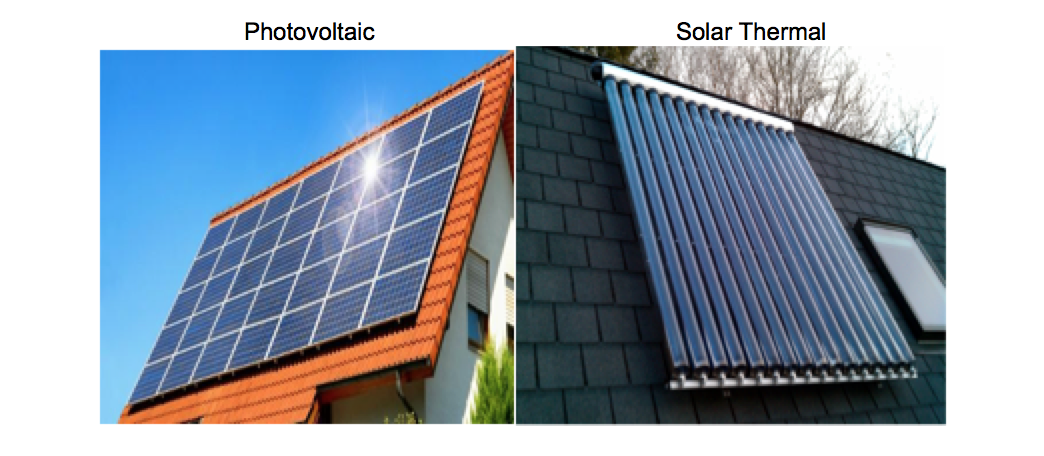
Figure 1
Through a technical analysis to compare photovoltaic and solar thermal, three main advantages of photovoltaic came to light. First, photovoltaic cells are more economically feasible, and the price of panels continues to decline (J. Nicodemus, personal communication, 2017). In the past year the NREL reported that commercial solar PV system cost fell nearly 15%. Secondly, photovoltaic panels are more aesthetically pleasing, benefitting campus architecture, an important aspect for campus architect Mary Wilford-Hunt. Generally the low architectural quality characterizing solar thermal pinpoints as one major reason for the low spread of the technology. As confirmed by examples of photovoltaic, the improvement of the architectural quality can increase the use of a solar technology even more than price (Probst, 2011). The third main advantage of photovoltaic panels is that it requires minimal maintenance. For optimal efficiency the panels should be cleaned twice a year (J. Nicodemus, personal communication, 2017), while rooftop solar thermal panels and water tanks need regular maintenance to operate at peak efficiency (Energy.gov, ). Because of the economic advantages, architectural benefits and low maintenance, photovoltaic panels are recommended as the best option for the Lafayette College feasibility study.
In order to fully realize the benefits of solar energy, Lafayette College must be able to calculate the returns on energy for photovoltaic panels. The main equation for returns on energy for photovoltaic panels is Mpv=. Where Mpv is the efficiency of photovoltaic panels, which is traditionally set at 15% (j. Nicodemus, personal communication, 2017). Epv is the energy produced by the photovoltaic panels and Esol is the energy from the solar resource (the sun). It is then derived that Esol=IsolApv, where Isol equals the kwh/m2day received in Easton, PA and Apv is the area of solar panel receiving energy from the sun (J. Nicodemus, personal communication, 2017). Maps.nrel records that the average annual Isol in Easton, PA is 4.43 kwh/m2day. Therefore the final equation for return on energy for photovoltaic solar energy is Mpv=, where Mpv= 15% and Isol= 4.43 kwh/m2day. The only unknown variable needed to calculate the return on energy for photovoltaic solar energy is the panel area that will be absorbing photons from the sun.
In order to fully optimize the returns on energy for photovoltaic solar panels it is important to angle the panel to optimize the amount of sunlight it receives. Photovoltaic panels work most efficiently when sunlight is hitting it perpendicularly. The optimal angle for photovoltaic solar panels is equal to the latitude of the location the panels are installed at. Crawley (2016) states, “it is generally accepted that the tilt angle that maximizes the annual energy collection is approximately equal to the latitude” (Pg. 53). In the case of Lafayette College the optimal angle for photovoltaic solar panels is approximately 40.7 degrees.
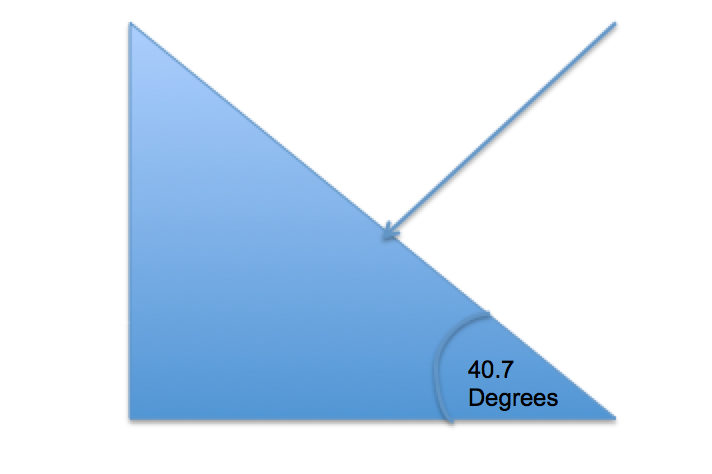
Figure 2
Another strategy used to determine the feasibility of installing solar panels at Lafayette College is to analyze efforts completed by other higher educational institutions. In 2014, Endicott College installed a solar canopy over a 255-space parking lot (Helmer, 2017). Endicott College is located in Massachusetts, experiencing a similar climate to Lafayette College. In fact, Endicott has an annual smaller total solar resource than that of Lafayette College (Maps.nrel). The college reported the installations generated up to 12 million kilowatt hours of power per year, about 10 percent of total campus energy use, at a price lower than the school pays for natural gas (Helmer, 2017). Furthermore the Association for the Advancement of Sustainability in Higher Education reported that they are aware of 330 institutions that have deployed more than 600 installations nationwide, with the total solar capacity in higher education at approximately 247,776 kilowatts. College campuses across the country have successfully implemented solar energy systems and we strongly believe Lafayette College can follow in the steps of the 330 institutions before them and become a model institution for solar energy.
Location Selection
Another important step in determining the optimal location where the photovoltaic solar panels should be installed. Four criteria were set in order to rate each potential location on its feasibility to house photovoltaic panels. The first criterion is that the location must allow the panels to be south facing. Photovoltaic panels receive the most amount of direct sunlight when they are south facing (J. Nicodemus, personal communication, 2017). Related to this, the second criterion is that the panels must be placed in a location where they can receive direct sunlight. A location where they would be blocked by trees or other buildings would not serve as an optimal location for photovoltaic solar panels. It is crucial to balance the system size and visibility while not sacrificing the solar resource (Pratt, 2013). The third criterion is that the building must have the load capacity to hold photovoltaic panels. A common photovoltaic panel weighs between 15 to 17 kg/m2, with the majority percentage of the weight coming from the glass followed by the aluminum frame (Crawley, 2016). In order to get details of load capacity for Lafayette College buildings our research team sat down with the Lafayette College Architect, Mary Wilford-Hunt. The final criterion is that the chosen building must allow the photovoltaic solar panels to be visible by pedestrians. As discussed is the Social Context section, visible solar energy is a top priority of our team. After analyzing several buildings on campus, three stood out.
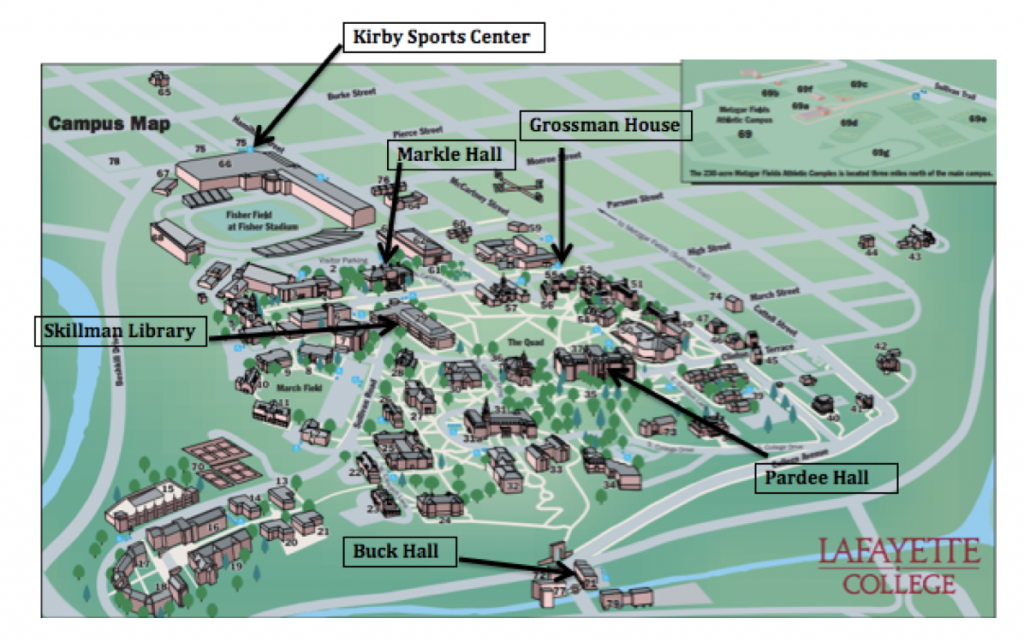
Figure 3: Map of Lafayette College’s campus with possible buildings labeled.
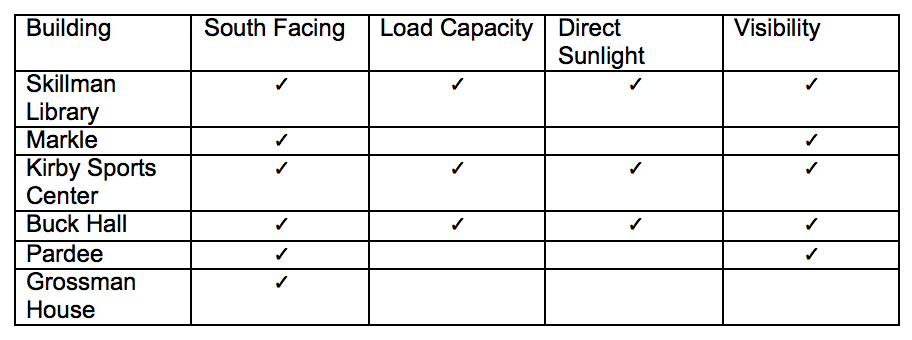
Figure 4
Figure 3, maps out six different potential locations across Lafayette Campus. The six are Skillman Library, Markle Hall, Kirby Sports Center, Buck Hall, Pardee Hall and Grossman House. As shown in the table above, three buildings meet all four criteria for being a location that can house photovoltaic solar panels. The three building are Skillman Library, Kirby Sports Center, and Buck Hall.
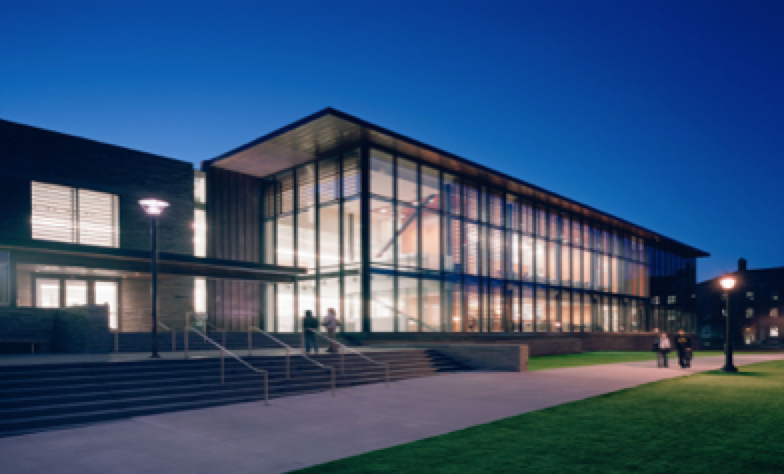
Figure 5: Front view of Skillman Library.
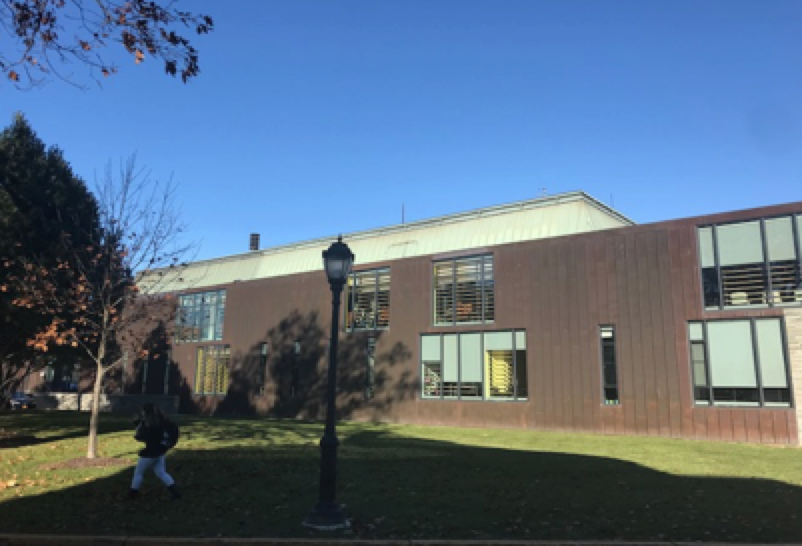
Figure 6: Back view of Skillman Library.
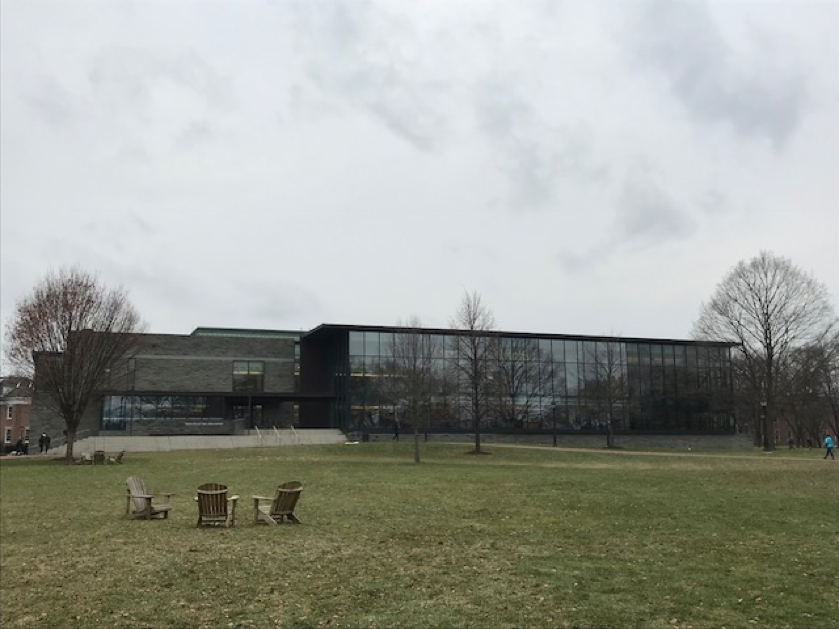
Figure 7: View of Skillman Library from steps of Farinon
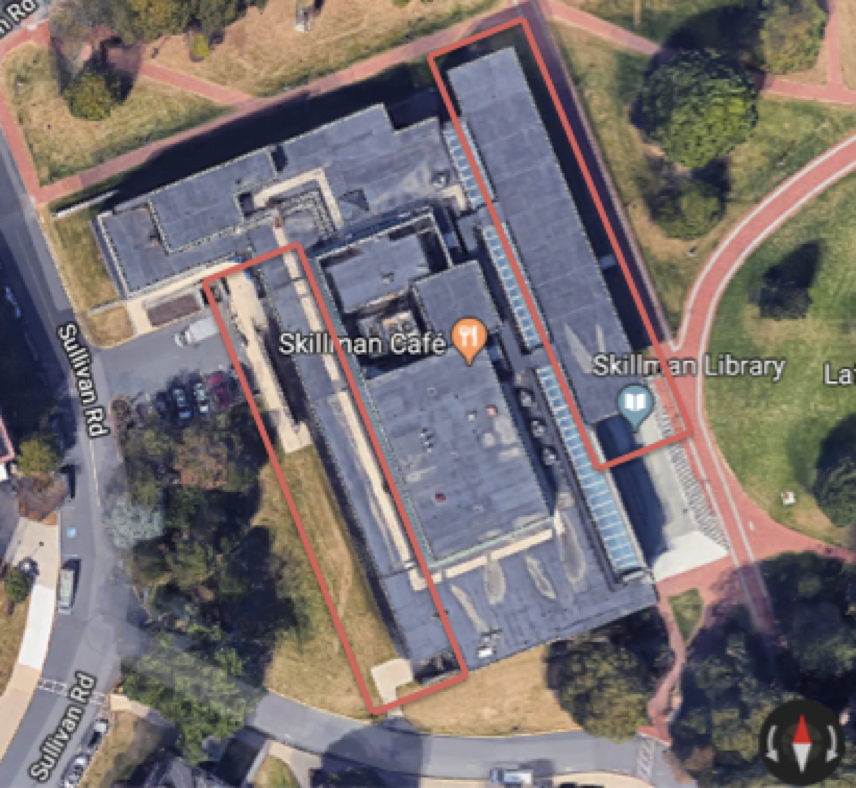
Figure 8: Aerial view of Skillman Library; Source: Google Maps
Skillman Library acts as the center point for the Lafayette Campus. It is one of the most popular buildings on campus and acts as a symbol for the college. Skillman Library does have the necessary load capacity to house photovoltaic panels (M.Wilford-Hunt, personal communication, 2017). Also due to its location on campus most students and visitors will be guaranteed to pass by the building daily. However the level of visibility for solar panels is in question. As shown the in figure 5, showing the front of the library, there is no clear perspective of the roof. Solar panels are placed at angles, which aids to their visibility on a flat roof. Figure 7, shows the perspective of Skillman from the steps of Farinon, where angled solar panels would be visible. In figure 6, showing the back of the library, there is improved visibility of the roof. Skillman does receive direct sunlight and is capable of hosting south facing solar panels at the recommended spots, outlined in figure 8.
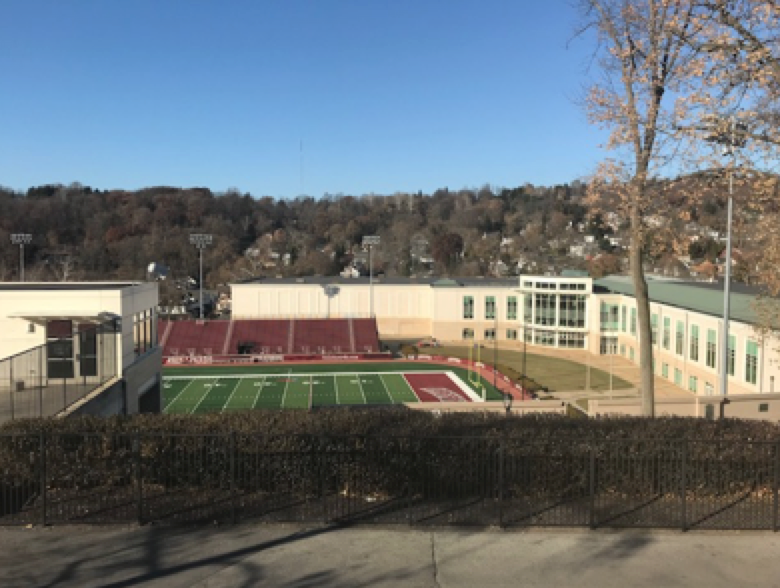
Figure 9: View of Kirby Sports Center.
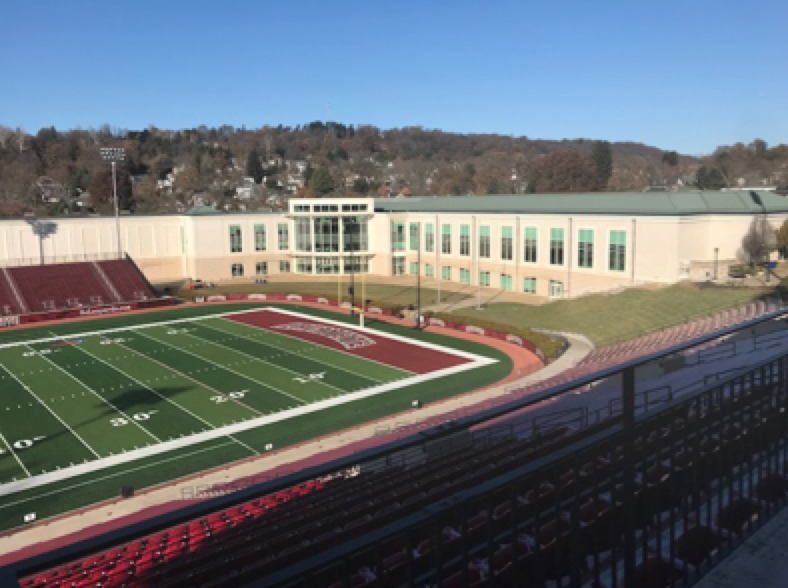
Figure 10: View of Kirby Sports Center.
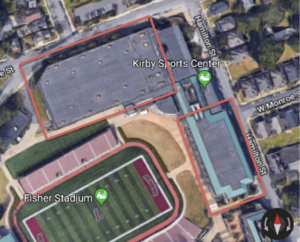
Figure 11: Aerial view of Kirby Sports Center; Source: Google Maps
The second building that met all four criteria is Kirby Sports Center. Kirby Sports Center is especially attractive because it will allow for visible solar energy that can be seen from football games and other populated athletic events at Fisher Field, as shown in figure 8. Also figure 7 shows the vantage point from the Markle parking deck, a common parking place for perspective students. Furthermore, Kirby Sports Center has the load capacity, no obstructions from direct sunlight and plenty of area to house solar panels. (M.Wilford-Hunt, personal communication, 2017). The outlined recommended housing space, shown in figure 11, will be visible to those in the stadium and from Markle Parking deck. As a large athletic facility, the Kirby Sports Center requires energy that could be harvested through the sun instead of burning fossil fuels.
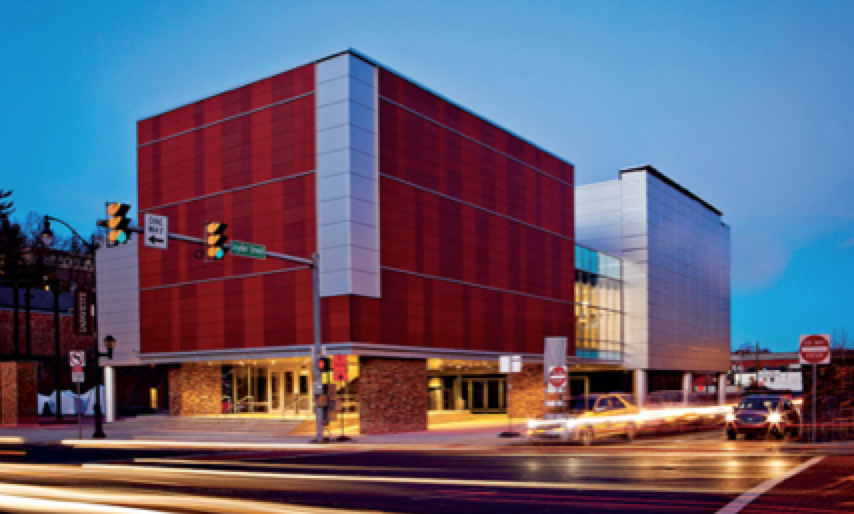
Figure 12: View of Buck Hall.
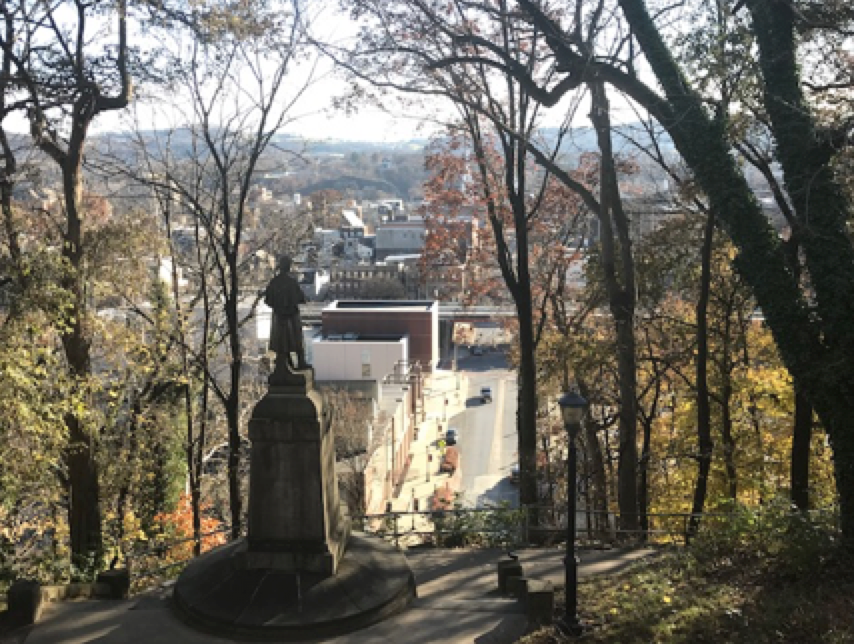
Figure 13: View of Buck Hall from Lafayette’s campus on College Hill.
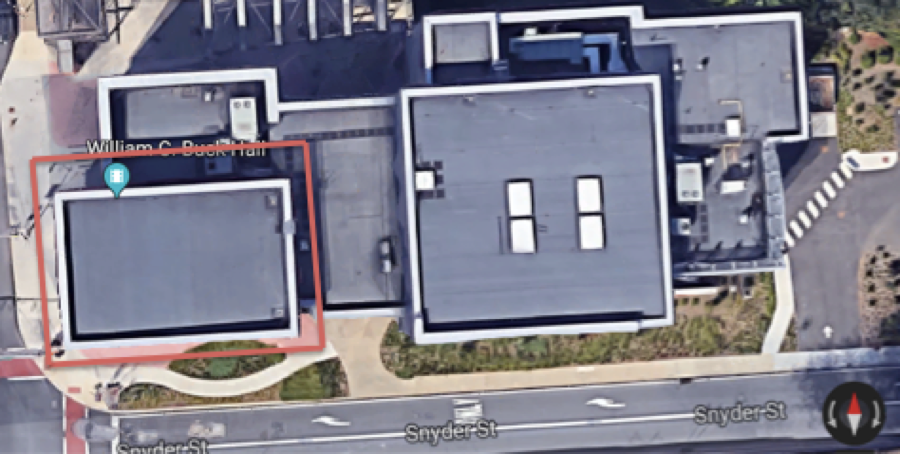
Figure 14: Aerial view of Buck Hall; Source: Google Maps
The third and final building that met all four criteria is Buck Hall. Located down the hill, Buck Hall acts as a welcoming entity for all those entering the Lafayette College Campus (Figure 9), allowing for visible solar energy that can achieve the goals stated in the Social Context section. From College Hill, the roof of Buck Hall is easily visible as one uses the stairs to access downtown Easton (Figure 10). This is especially notable because of future talks of implementing an elevator, only increasing the visibility of the Buck Hall’s roof. Furthermore, it was discovered that Buck Hall is actually constructed to hold solar panels, but due to a lack of budget the plan never went through (M.Wilford-Hunt, personal communication, 2017). Therefore, Buck Hall meets all four criteria as a suitable building to house solar energy.
Conclusion
Through the technical analysis performed, the research team analyzed different solar energy methods, how to fully optimize solar energy and where Lafayette Campus should house solar panels. The technical analysis performed is a start to Lafayette College implementing solar energy on campus, however there are some next steps that would be beneficial to the further the process. First would be to exactly define the area of photovoltaic solar panels that would be installed. By doing so, Lafayette College would have more accurate metrics on how much energy they should expect from implementing photovoltaic solar panels. This is an important step in the process, because real metrics to show the returns on energy expected will aid in getting administration approval for a solar energy project. Further analysis of the buildings selected for optimal locations for photovoltaic solar panels could also be beneficial to the study. It will be crucial to place solar panels in visible areas of the roof of the selected building. Through a physical analysis of the roof more information can be collected on the area of photovoltaic panels that can be installed.
As a prestigious institution, Lafayette College is in the unique position to implement renewable energy on campus in terms of solar energy. In terms of the technical context, Lafayette College stands in a great position to move forward in the process of installing solar panels on campus. In the 21st century, photovoltaic technology has demonstrated the maturity to become a major source of power for the world (Crawley, 2016). With prime solar housing locations, along with advanced solar technology, Lafayette College is ready to continue the process to implement solar energy systems on campus.
Next Section: Economic Analysis